Research
Cavity-enhanced 2D infrared spectroscopy
The goal of my MSCA fellowship is to build a cavity-enhanced two-dimensional infrared (CE-2DIR) spectrometer and to apply it to detection of complex mixtures of volatile organic compounds (VOCs). This will be done by resonantly enhancing multiple optical frequency combs inside an optical cavity for improved concentration sensitivity and nonlinear signal selectivity.
Detection of trace amounts of VOCs in multi-species mixtures is important for many purposes, ranging from basic science, through analysis of complex chemical environments such as flames, to breath analysis for medical diagnostics. The most commonly used methods for detection of VOCs are based on mass spectrometry and are encumbered with several important disadvantages that motivate development of alternative solutions [1]. Laser spectroscopy solutions offer sub-second time resolution, excellent accuracy and detection limits from part-per-million (ppm) to part-per-billion (ppb) in a compact form. However, they are generally limited to detecting a single compound at once due to single-wavelength operation. To overcome this limitation, a significant effort has been put forward in developing frequency comb-based spectrometers, which use “million stable lasers at once” in a single beam for multi-species detection. Unfortunately, nature presents a fundamental difficulty for linear spectroscopy by making spectra of different compounds overlap (see fig. 1)
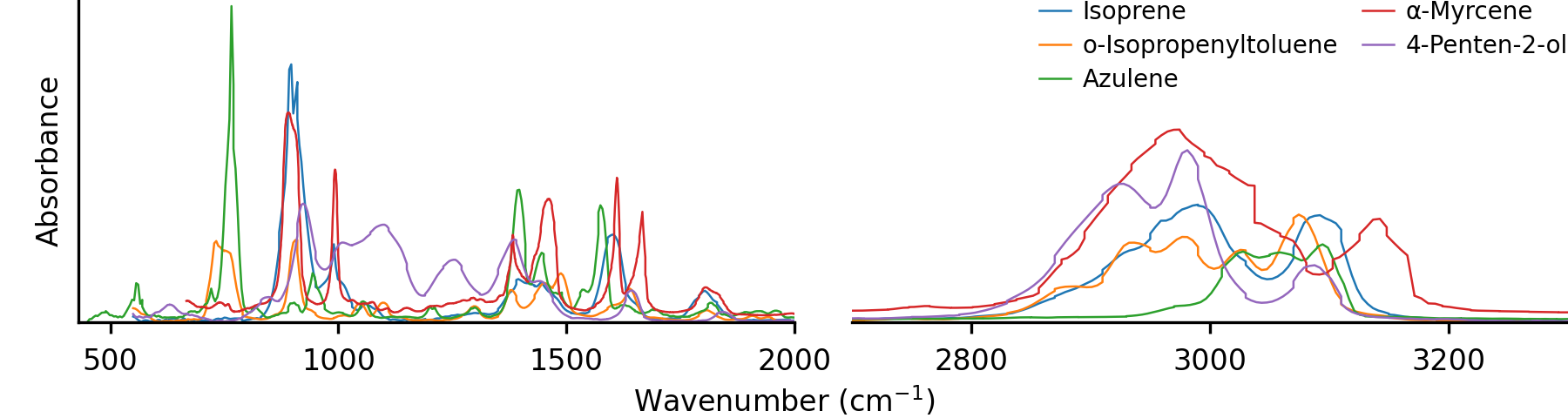
Coherent third-order (nonlinear) spectroscopies using ultrashort pulses, such as transient absorption spectroscopy (TAS) and 2D spectroscopy, are powerful tools for studying both structure and dynamics. In a common experimental arrangement, three ultrashort pulses (~100 fs) are made to interact with the sample in sequence, and the molecular response is recorded as a function of delays between pulses. In a simplified picture, the first two (pump, dump) pulses excite some vibrational mode of the molecule (e.g. C-H stretch), putting a fraction of the sample in an excited state, and the last one probes the response of the molecule in the excited state. As a result, two-dimensional spectra are obtained reporting on the correlations between excitations of different bonds in the molecule. These correlations strongly depend on the structure of the molecule as whole and not only on the particular bonds that are excited and so 2D spectroscopy provides much greater selectivity than linear spectroscopy.
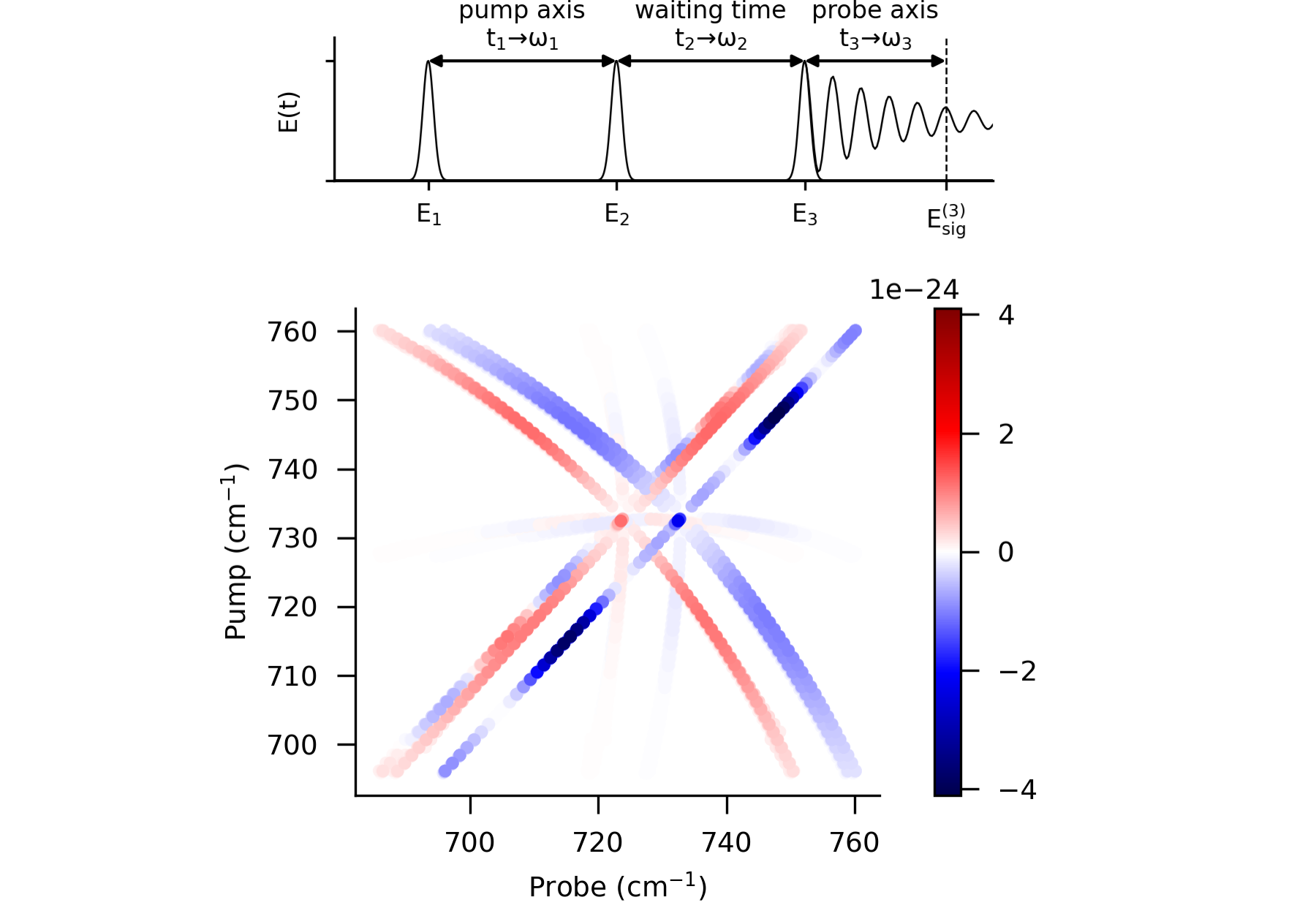
To obtain the necessary sensitivity for detection of trace amounts of gases with 2D spectroscopy, we will use optical frequency combs resonantly enhanced inside an optical cavity. Optical frequency combs are generated by phase-stable lasers emitting trains of ultrashort pulses. In frequency domain, such pulse train corresponds to a comb of narrow laser lines (see fig. 3). Optical cavities posses similar spectrum, consisting of a comb of almost-equidistant resonances, and are commonly used in spectroscopy to increase the optical path length or equivalently to build up power inside the resonator. When the resonator contains an absorbing gas sample, this results in increase of absorption and lower detection limits. We will expand on the methods recently developed for cavity-enhanced transient absorption spectroscopy [2, 3, 4] and the scheme to use higher-order transverse cavity modes for cavity enhancement of nonlinear signals [5].
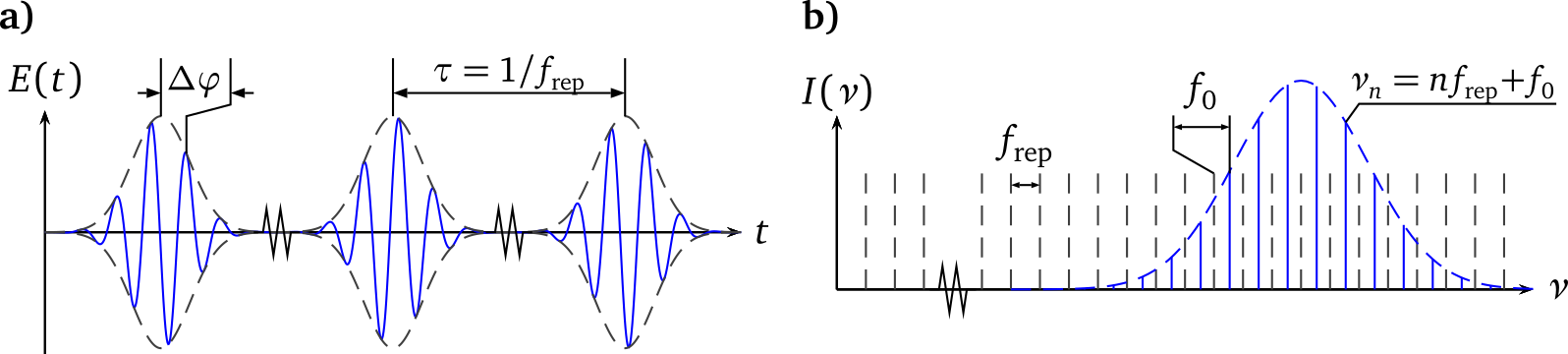
This project receives funding from the European Union’s Horizon 2020 research and innovation programme under the Marie Skłodowska-Curie grant agreement No 101028278.
References
- [1] B. Henderson et al., Applied Physics B 124, 161 (2018). doi: 10.1007/s00340-018-7030-x
- [2] Y. Chen, M. C. Silfies, G. Kowzan. J. M. Bautista, T. K. Allison. Applied Physics B 125, 81 (2019). doi: 10.1007/s00340-019-7191-2
- [3] M. Silfies, G. Kowzan, Y. Chen, N. Lewis, R. Hou. R. Baehre, T. Gross, T. K. Allison. Optics Letters 45, 2123–2126 (2020). doi: 10.1364/OL.389412
- [4] M. C. Silfies, G. Kowzan, N. Lewis, T. K. Allison. Physical Chemistry Chemical Physics 23, 9743-9752 (2021). doi: 10.1039/D1CP00631B
- [5] T. K. Allison. Journal of Physics B 50, 044004 (2017). doi: 10.1088/1361-6455/50/4/044004